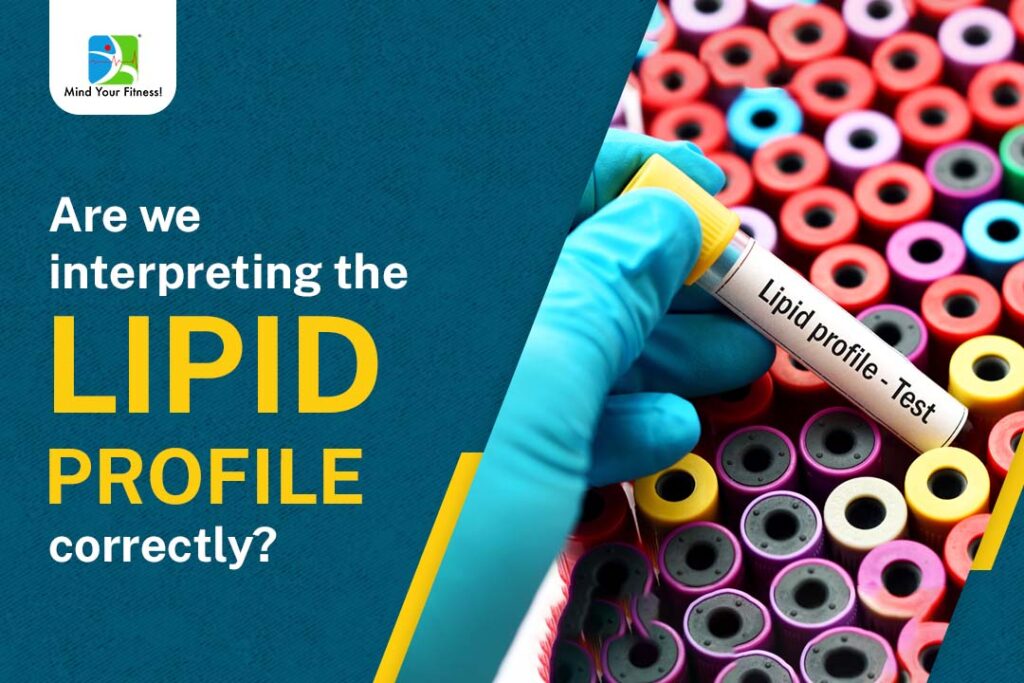
Interpreting a lipid profile correctly can be quite daunting. With new studies indicating how total cholesterol values cannot be used in isolation to gauge heart disease risk, how should a lipid profile be analysed?
What does the Lipid Profile measure?
Standard fasting blood tests for cholesterol and lipid profiles will include values for total cholesterol, HDL cholesterol, LDL/VLDL cholesterol and triglycerides.
Let’s understand the individual components.
Cholesterol is a fatty substance that is necessary for building cells and producing hormones. In the body, its produced in your liver but we can also get it from the animal based foods we eat, like meat, eggs, poultry, and dairy products. When cholesterol is consumed in the diet, the liver produces less cholesterol. This is a feedback mechanism in those with a normal metabolism. This mechanism may not work in those with diabetes or genetic disorders. Total cholesterol (TC) increases when there is a rise in LDL or HDL or both.
Triglycerides (TG) are the fats that are transported in your blood (fasted state) and stored in your fat cells.
Lipoproteins (vehicles) are carriers of triglycerides and cholesterol (passengers). The main types reflected in your lipid profile are HDL, LDL, and VLDL.
HDL stands for high-density lipoproteins (HDL). It is referred to as the “good” cholesterol because it carries cholesterol from other parts of your body back to your liver. Studies do show lower risk of heart disease with higher HDL levels. Therefore, the TC/HDL and LDL/HDL cholesterol ratios are also relevant risk markers.
Low-density lipoproteins (LDL) & very low-density lipoproteins (VLDL) mainly transport cholesterol and triglycerides from the liver to the organs. They are the ones that can lodge in your arteries, that’s why they are usually referred to as “bad” cholesterol.
Before we call them bad, let’s understand their composition and how they contribute to disease risk.
Lipoprotein refers to lipid + protein.
Different lipoproteins carry varying amounts of triglycerides and cholesterol which are the lipid parts. VLDL particles are richer in triglycerides whereas LDL particles are richer in cholesterol. VLDL becomes LDL in circulation.
Particle size:
VLDL particles are larger than LDL particles.
Interestingly, those on high carbohydrate diets tend to be associated with larger, TG-rich VLDL1, and those with diets low in carbohydrates and higher in fat tend to have smaller, but higher in cholesterol ester—VLDL2. (1)
If the triglycerides are high, VLDL will convert into smaller dense LDL. Smaller particles are more likely to clog arteries. (2)
Therefore, it can be assumed that when the triglycerides are high, the risk of atherosclerosis is higher. Lower triglycerides are more likely to form the large buoyant, less atherogenic LDL.
Small dense LDL are highly atherogenic as a result of their low binding affinity for the LDL receptor, they remain in circulation longer and are more prone to oxidation. (14)
In dyslipidemic patients with insulin resistance and visceral obesity, HDL subclass distribution is altered to contain an increased concentration of small, dense HDL (HDL3 subclass) and decreased large HDL particles (HDL2 subclass). HDL subclass distribution is associated with the progression of cardiovascular disease. Studies demonstrate an inverse relationship between coronary heart disease risk and HDL2b levels and a positive relationship to HDL3c and HDL3b levels. (1)
Particle number:
The main protein (apoprotein) associated with VLDL and LDL is called Apolipoprotein (apoB) or apoB100.
The LDL in your lipid profile actually reflects the cholesterol component of these particles (passenger). It is an indirect marker of the heart disease risk. It assumes that more passengers means more vehicles.
ApoB can be measured but is never a part of routine lipid profiles. Studies now show that measuring apoB is a more accurate marker of heart disease risk compared to total cholesterol and LDL. It’s a marker of the number of LDL particles in circulation.
ApoB concentrations measure total LDL, intermediate density lipoproteins (IDL), VLDL, and lipoprotein(a) (Lp(a)) particle concentrations because each of these particles contain exactly one apoB100 molecule. Prospectively, it has been shown to improve risk prediction for cardiovascular events after controlling for traditional risk factors.
ApoB concentrations of < 90 mg/dL (< 80 in very high risk patients) is the target goal proposed as by National Lipid Association in patients treated for cholesterol. (2)
Higher the number of vehicles, higher is the chance of a jam. More passengers may not cause jams as there could be fewer vehicles with each of them packing more passengers.
Discordance and Genetic variants:
Higher triglycerides are more likely associated with small dense atherogenic LDL particles and subsequently higher apoB levels.
Sometimes we see genetic variants with high triglycerides/normal apoB levels and also normal triglycerides/high apoB levels. Therefore, between the two, apoB would be a more accurate marker of the total atherogenic particles in circulation.
In patients with apoB mutations, hepatic VLDL secretion is impaired and often leads to fatty liver (steatosis). The extent of steatosis is related to the size and number of VLDL particles produced within this patient population. Depending on the particular apoB mutation, either smaller particles carrying lower than normal amounts of TG or a smaller number of particles carrying higher than normal amounts of TG are secreted. These two types of particles display different kinetics in the blood compartment, and cause different types of dyslipidemia. (1)
Also, if your LDL cholesterol is high, but your apoB is normal (called discordance), then you probably have less to worry about.
Individuals with discordantly high apoB to LDL levels carry a higher risk of atherosclerotic disease compared with those with average or discordantly low apoB to LD-cholesterol. (3)
Risk factors that accelerate Atherosclerosis:
Several factors contribute to atherosclerosis of which higher LDL particles in circulation is only one of the factors. First of all, we should pay attention to lipid levels before infancy since fetuses born to hypercholesterolemic mothers will have fatty streaks, and the development of atheroma is accelerated in the children of such pregnancies (11).
A. Oxidative stress and inflammatory processes are of major importance in atherogenesis.
- Oxidative stress causes increased formation of oxidised LDL (Ox-LDL). In turn, this stimulates macrophage cholesterol accumulation and foam cell formation, the hallmark of early atherosclerosis. Under oxidative stress, plasma lipoproteins invade the arterial wall, where they are exposed to atherogenic modifications. A native LDL cannot exert atherogenic mechanisms in vitro which implicate that to be pathogenic it must have been modified, explaining oxidative damage as the starting point. Polyunsaturated fatty acids (PUFA’s) in the LDL particles are more prone to oxidation as compared to monounsaturated fatty acids (MUFA’s) (12). So the type of fats/oils in the diet matters.
- Protection of LDL against oxidation by antioxidants that can act directly on the LDL, or indirectly on the cellular oxidative machinery, or conversion of Ox-LDL to a non-atherogenic particle by HDL-associated paraoxonase (PON-1), can contribute to attenuation of atherosclerosis. High-density lipoproteins, endogenous apolipoprotein E (ApoE), and HDL associated ApoA-I apoproteins promote efflux of surplus cholesterol from the macrophage. This efflux is the primary line of defence to protect against the progression of macrophage to foam cells. Antioxidants scavenge free radicals and reduce oxidative stress. Antioxidants can be synthesized by a cell itself or can be taken exogenously. Antioxidants synthesised by a cell include glutathione, uric acid, ceruloplasmin, ferritin, transferrin, or lactoferrin, whereas vitamin E, vitamin C, flavonoids, and carotenoids come from the diet.
B. Endothelium injury
Focal response to chronic hemodynamic stress eg., hypertension, dylipidemia or other forms of injury may functionally alter the endothelium and cause greater influx of plasma lipoproteins and smooth muscle cell proliferation, resulting in increased synthesis of proteoglycans with altered characteristics. Enhanced binding of apoB containing lipoproteins to proteoglycans under these conditions sets the stage for the development of atherosclerosis (13). So maintaining the overall integrity of the blood vessels is important in the prevention of atherogenesis.
What increases Apo B levels?
Genetic tendency, being overweight, obesity and defective apoB metabolism
can cause higher apoB levels. (2)
Some people are more sensitive to mild increases of saturated fats (SFA) in the diet due to their genetic makeup (hyper responders). It translates into higher apoB levels or higher small dense LDL (LDL phenotype B). (10)
Individuals with high apoB, irrespective of LDL, tend to have higher consumption of SFA and carbohydrates. (3)
Eating carbohydrates in excess of requirement can lead to excessive amounts of triglycerides in blood. Glucose formed from digestion of carbohydrates gets converted to triglycerides in the liver. Eating a high carbohydrate/high fat diet accelerates this. High triglycerides could also mean more apoB in circulation. (4)
Alcohol does the same.
LDL increases on low carb (LC)/ketogenic diets (KD): This is normal!
1. It is noteworthy that ketogenesis and cholesterol synthesis share a common pathway whereby acetyl CoA is converted to acetoacetyl-CoA and then β-Hydroxy β-methylglutaryl-CoA (HMG-CoA) by thiolase and HMG-CoA synthase, respectively.
In the synthesis of cholesterol, HMG-CoA is converted to mevalonate by HMG-CoA reductase, whereas in the synthesis of ketones HMG-CoA is converted to acetoacetate by HMG-CoA lyase.
Although ketone synthesis occurs exclusively in the mitochondria and cholesterol synthesis in the cytoplasm, it is possible for some acetoacetate generated during ketogenesis to diffuse out of the mitochondria and be converted to acetoacetyl-CoA in the cytosol via the action of acetoacetyl-CoA synthetase.
Thus, the higher flux of fatty acids and ketogenesis specially seen in LC athletes in the context of overall high energy expenditures could contribute to increased endogenous synthesis of cholesterol by enhancing the cytosolic substrate pool.
LC athletes who adopt a ketogenic diet may experience an expansion of their endogenous cholesterol pool during the adaptation phase of the diet, after which they maintain greater circulating cholesterol levels. (5)
2. Previous studies have shown that increases in LDL1(larger LDL) resulting from substitution of dietary saturated fat for carbohydrate or unsaturated fat are due primarily to increases in large less atherogenic cholesterol-enriched LDL, with minimal changes in small, dense LDL particles and apoB. (2)
3. Saturated fats are known to raise the levels of LDL and HDL cholesterol.So total cholesterol tends to be higher in those following ketogenic diets. Since both increase, the LDL/HDL ratio also improves lowering risk.
On a LC diet, HDL tends to go up and triglycerides down, while total and LDL cholesterol tend to stay the same or increase marginally. LDL particle size tends to increase and LDL particle number tends to go down. (2)
All good things!
4. The Friedewald equation named after William Friedewald, has been used for decades around the world to calculate LDL-C. In a paper published in 2001, Wang and colleagues described a patient with a low TG value of approximately 50 mg/dL, a high total cholesterol level, and a discrepant LDL level. The LDL level using the Friedewald calculation turned out to be much higher than the LDL level using direct measurement.
The authors suggested for the first time that in the presence of low TG and high total cholesterol levels, the LDL level should be measured directly instead of using the Friedewald calculation. These results were later confirmed in a paper published 2008 by Iranian investigators showing that with low concentrations of TG, the Friedwald equation may overestimate LDLlevels to such a degree that may affect clinical decision-making.
This could have practical implications for people who follow a ketogenic lifestyle. One of the first thing that happens during carbohydrate restriction is that blood levels of TG drop, often dramatically. (6)
This could lead to an overestimation of LDL levels in the lipid profile.
Who’s the real culprit? Saturated fat or Carbohydrates?
Smaller LDL particles are not reduced by saturated fat restriction in the majority of individuals.
It is important to distinguish between dietary saturated fat and circulating SFA. Whereas several reports show no association between increased intake of SFA and risk for chronic disease, individuals with higher circulating levels of even-chain SFA (particularly palmitate) have increased risk of disease.
Notably, the amount of circulating SFA in blood is not related to saturated fat intake from the diet but instead tends to track more closely with dietary carbohydrate intake. (7)
Even those not willing to fully acquit saturated fat from the role of the heart’s villain, accept that carbohydrate consumption should be moderated.
Studies like MESA and EPIC, which have shown worse metabolic and cardiovascular outcomes with higher blood levels of even-chain SFAs, implicate carbohydrates because plasma levels of even-chain SFAs correlate more strongly with drivers of de-novo lipogenesis, including alcohol, soft drinks, and potatoes, than with dietary sources of SFA such as meat, butter, or cheese. If you compare saturated fats to carbohydrates, , they have a fairly neutral effect on blood lipids. (8)
Switching dietary intake to a high carbohydrate/low fat diet has been associated with an increase in plasma apoB concentration. The effect of these dietary changes was reported to be mediated via an increased flux of carbohydrate to the liver, which led to upregulated de-novo lipogenesis and the subsequent production of apoB. This consequently also decreased HDL-cholesterol levels. (3)
All bad things!
Diet-heart hypothesis
The concept that saturated fat causes cardiovascular disease by raising serum cholesterol is called the ‘diet-heart hypothesis’, a highly influential idea that has been a lynchpin of nutrition policy for some 60 years.
This hypothesis remains today a foundation of public health policy, with nearly all dietary guidelines worldwide recommending a cap on saturated fat consumption as a primary measure of protection against heart disease.
Over the past 12 years, however, there has been a major shift in scholarly understanding of these fats, with now >20 review papers, by independent teams of scientists, on the whole concluding that saturated fats have no effect on major cardiovascular outcomes, including heart attacks, strokes or cardiovascular mortality, or total mortality.
Data from the largest-ever epidemiological cohort study ever conducted, called Prospective Urban Rural Epidemiology (PURE), provides this type of contradictory evidence regarding the diet-heart hypothesis.
PURE followed individuals aged 35–70 years, from 2003 to 2013, in 18 countries with a median follow-up of 7 4 years. The PURE investigators found that saturated fat was not associated with risk of myocardial infarction or cardiovascular disease mortality and was significantly associated with lower total mortality as well as lower risk of stroke.
This last finding, on stroke, is particularly significant, as it is consistent with other observational studies, and saturated fat is the only type of fat found to have a positive effect on this important cardiovascular health outcome.
Further, nine reviews of the observational data conducted since 2010 have found no significant associations between the consumption of these fats and coronary heart disease. (9)
Takeaway:
Don’t blame the butter for what the bread did! Individual risk assessment and diet customisation is key to maintaining good metabolic profiles and reduction in the risk of heart disease.
REFERENCES
1. German JB, Smilowitz JT, Zivkovic AM. Lipoproteins: When size really matters. Curr Opin Colloid Interface Sci. 2006 Jun;11(2-3):171-183. https://www.ncbi.nlm.nih.gov/pmc/articles/PMC2893739/
2. Dansinger, M., Williams, P.T., Superko, H.R. et al. Effects of weight change on apolipoprotein B-containing emerging atherosclerotic cardiovascular disease (ASCVD) risk factors. Lipids Health Dis 18, 154 (2019). https://lipidworld.biomedcentral.com/articles/10.1186/s12944-019-1094-4
3. Mazidi M, Webb RJ, George ES, Shekoohi N, Lovegrove JA, Davies IG. Nutrient patterns are associated with discordant apoB and LDL: a population-based analysis. Br J Nutr. 2022 Aug 28;128(4):712-720. https://www.ncbi.nlm.nih.gov/pmc/articles/PMC9346615/
4. Campos H, Blijlevens E, McNamara JR, Ordovas JM, Posner BM, Wilson PW, Castelli WP, Schaefer EJ. LDL particle size distribution. Results from the Framingham Offspring Study. Arterioscler Thromb. 1992 Dec;12(12):1410-9. https://pubmed.ncbi.nlm.nih.gov/1450174/
5. Creighton BC, Hyde PN, Maresh CM, Kraemer WJ, Phinney SD, Volek JS. Paradox of hypercholesterolaemia in highly trained, keto-adapted athletes. BMJ Open Sport Exerc Med. 2018 Oct 4;4(1). https://www.ncbi.nlm.nih.gov/pmc/articles/PMC6173254/
6. Wang TY, Haddad M, Wang TS. Low triglyceride levels affect calculation of low-density lipoprotein cholesterol values. Arch Pathol Lab Med. 2001 Mar;125(3):404-5.
7. Arne Astrup, Faidon Magkos, Dennis M. Bier, J. Thomas Brenna, Marcia C. de Oliveira Otto, James O. Hill, Janet C. King, Andrew Mente, Jose M. Ordovas, Jeff S. Volek, Salim Yusuf, Ronald M. Krauss, Saturated Fats and Health: A Reassessment and Proposal for Food-Based Recommendations: JACC State-of-the-Art Review, Journal of the American College of Cardiology, Volume 76, Issue 7,2020,Pages 844-857. https://www.sciencedirect.com/science/article/pii/S0735109720356874
8. Tricia Ward. Saturated Fat and CAD: It’s Complicated. https://www.medscape.com/viewarticle/839360#vp_2?form=fpf
9. Teicholz N. A short history of saturated fat: the making and unmaking of a scientific consensus. Curr Opin Endocrinol Diabetes Obes. 2023 Feb 1;30(1):65-71. https://www.ncbi.nlm.nih.gov/pmc/articles/PMC9794145/
10. Chiu S, Williams PT, Krauss RM (2017) Effects of a very high saturated fat diet on LDL particles in adults with atherogenic dyslipidemia: A randomized controlled trial. PLOS ONE 12(2). https://journals.plos.org/plosone/article?id=10.1371/journal.pone.0170664
11. Higashi, Y. Endothelial Function in Dyslipidemia: Roles of LDL-Cholesterol, HDL-Cholesterol and Triglycerides. Cells 2023, 12, 1293. https://www.mdpi.com/2073-4409/12/9/1293
12. Khatana C, Saini NK, Chakrabarti S, Saini V, Sharma A, Saini RV, Saini AK. Mechanistic Insights into the Oxidized Low-Density Lipoprotein-Induced Atherosclerosis. Oxid Med Cell Longev. 2020 Sep 15;2020:5245308. https://www.ncbi.nlm.nih.gov/pmc/articles/PMC7512065/
13. Srinivasan SR, Radhakrishnamurthy B, Vijayagopal P, Berenson GS. Proteoglycans, lipoproteins, and atherosclerosis. Adv Exp Med Biol. 1991;285:373-81. https://pubmed.ncbi.nlm.nih.gov/1858569/
14. Chapman MJ, Guérin M, Bruckert E. Atherogenic, dense low-density lipoproteins. Pathophysiology and new therapeutic approaches. Eur Heart J. 1998 Feb;19. https://pubmed.ncbi.nlm.nih.gov/9519339/
~Shweta Bhatia, Registered Dietitian